Megan Kennedy
Department of Geology
The College of Wooster
Disclaimer: This material is based upon work supported by the National Science Foundation under Grant No. 9910805. Any opinions, findings, and conclusions or recommendations expressed in this material are those of the author and do not necessarily reflect the views of the National Science Foundation.
<– Return to main pages list
Navigation
Intro to Calving Glaciers
What is a calving glacier?
When a glacier experiences calving, large pieces of the ice break off at the margin, usually during the retreating stage in the glacial life cycle. Specifically, tidewater calving glaciers are those whose margin, or terminus, ends into a body of water (Figure 1). When calving occurs, these broken pieces of ice fall into the water and become icebergs. Calving is the most efficient way for tidewater glaciers to experience a loss of their ice mass, as well as for the world’s oceans to gain more water through the melting of icebergs (Van der Veen, 1997).
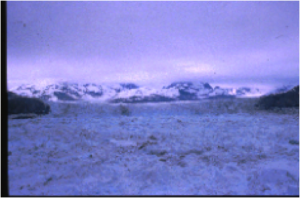
When do tidewater glaciers experience calving?
There are two types of controls on tidewater glacier advance and retreat and are therefore important in governing when and to what extent a glacier calves. These are climatic and non-climatic controls. Climatic controls drive the glacial system on a global scale and most often serve as the basis for wide-spread glacial advance or retreat. Of these, temperature and precipitation are the most predominant. Mercer (1961) described two climatic variables that are crucial to a glacier’s lifecycle (Figure 2). First, the equilibrium line altitude (ELA) is what separates the zone of accumulation (where snow falls and is compacted into ice) from the zone of ablation (where melting and calving occur). Glaciers begin to calve and retreat when ablation below the ELA is greater than accumulation above the ELA and is therefore dependent upon climate. Second, the accumulation area ratio (AAR) is the point above or below which a glacier will advance or retreat, respectively. It is a ratio of the glacier’s accumulation area to the glacier’s total area. When the AAR drops below 0.6, retreat and calving occur (Powell et al., 1991). These two variables maintain a complex relationship that serves as the basis for the climatic controls on glacial advance and retreat.
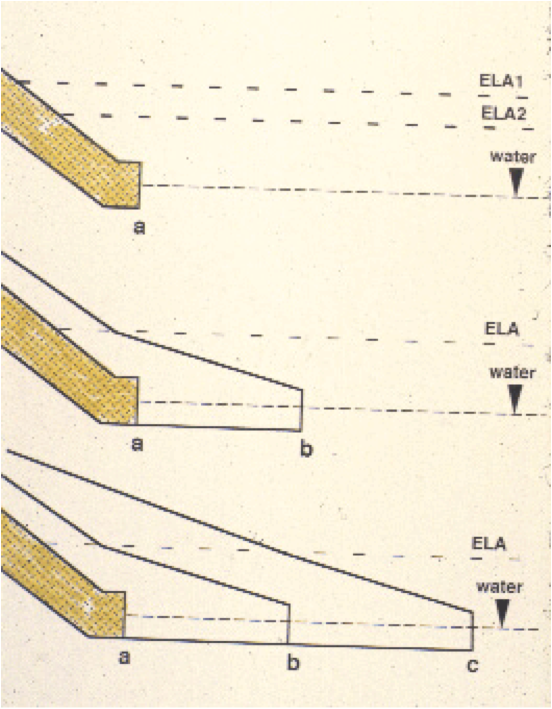
While non-calving glacial cycles are driven primarily by changes in climate, tidewater glaciers are also forced by a number of other factors in the system. In addition to climatic controls on calving, they must also respond to non-climatic conditions in and around the fjord in which they terminate. Some tidewater calving glaciers might respond equally to these controls as they do to climatic controls (Mann, 1986).
The first of these is the water depth in the fjord (Figure 3). As a glacier advances, it creates a moraine shoal below sea level, which is full of sediment and debris at the front of its margin. This pile of sediment acts as a support for the front of the ice as long as the glacier continues to advance. However, when the glacier begins its retreat (often dictated by climatic controls explained above), the moraine shoal stays grounded in place, and the terminus is over deeper water. Tidewater glaciers are known to experience rapid retreat and linear calving rates as the fjord water deepens because of a loss of stability at the margin (Figure 4). One key result of this loss of stability is the formation and detachment of icebergs due to crevassing throughout the ice; hence, calving begins and drives the system back further.
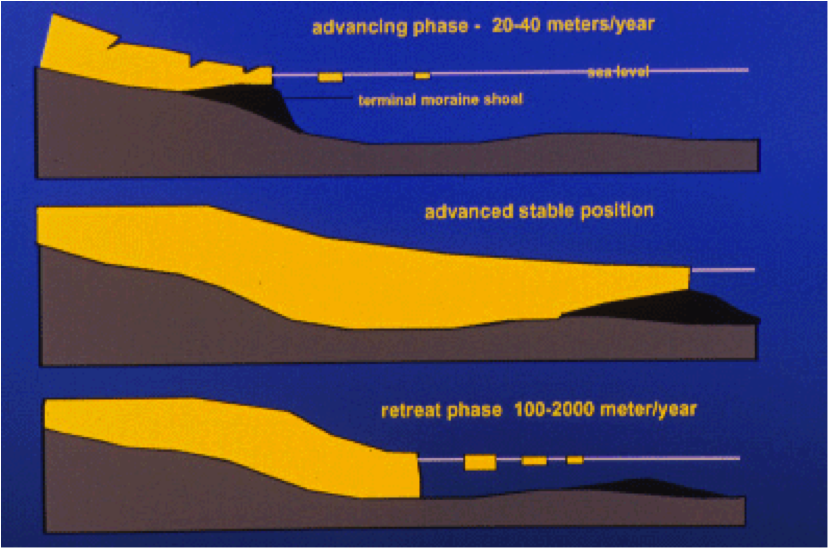
Another non-climatic influence on calving rates is ice thickness at the terminus. Thicker ice is also a sign of lesser stability and greater stresses at the calving front (Warren, 1992). A linear relationship has been found between calving rate and both ice thickness and water depth, as seen in figure 4 (Van der Veen, 1995).
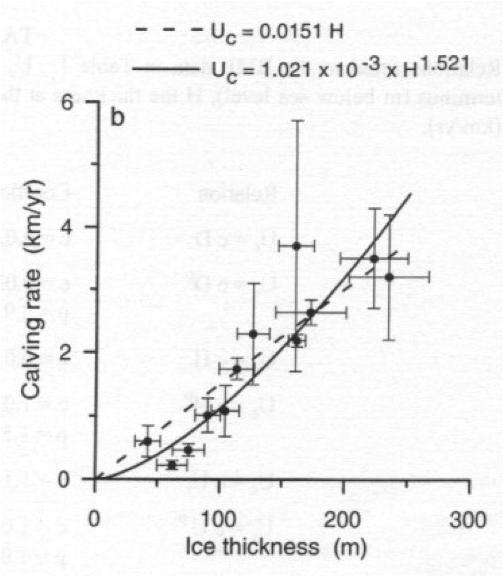
Finally, fjord geometry is an equally influential non-climatic factor in controlling tidewater glacier calving (Figure 5). As fjord width increases, the calving margin also widens, causing an increase in the surface area of the ice (Mercer, 1961). Again, this leads to lesser stability and greater stresses at the terminus, and retreat rate due to calving increases. Fjord geometry has been documented to quicken calving rates of tidewater glaciers so much that once the glacier reaches a certain point in its rapid retreat phase, it is no longer affected by climatic controls until a more narrow, stabilizing section of the fjord is reached (Mercer, 1961).
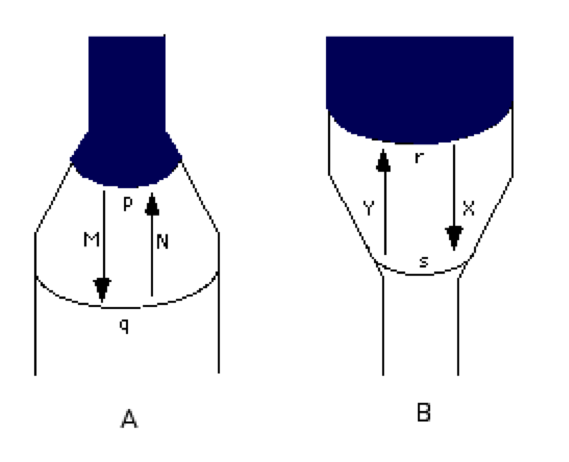
Do all calving glaciers behave the same?
Several attempts have been made to quantitatively determine a “universal calving law” that can be applied to any calving tidewater glacier in the world (i.e. Brown et al., 1982, Venteris, 1999). While numerical models have been formulated for individual glaciers and regions of glaciers, this hypothetical universal law is unlikely to exist because of the different climatic and non-climatic mechanisms that control calving rates.
One question that remains to be answered is whether calving depends on glacial retreat, or vice versa (Venteris, 1999). Some researchers agree that the linear relationship between calving rate and water depth (Figure 4) leads to the assumption that calving rates drive glacial retreat (Brown et al., 1986, Meier, 1994). However, others have stated that retreat drives calving rate because of the observation that calving occurs faster when the terminus position retreats into deeper water (Van der Veen, 1996). This relationship is unclear to date, and further study is needed to fully understand this aspect of tidewater glaciers.
Columbia Glacier
What is the importance of studying Columbia Glacier?
Among the world’s most studied tidewater glaciers is Columbia Glacier, located in Prince William Sound just west of Valdez, Alaska (Figure 6). It is well documented to have been experiencing calving and rapid retreat up its fjord since 1982. The water depth and fjord geometry at Columbia Glacier has allowed for its continued retreat to date, even though its AAR is above the equilibrium level of 0.6 required for it to begin advancing again (Lapham, 2001). Thus, it is apparent that non-climatic variables currently make more of an impact on the calving behavior of this glacier than do climatic variables, and it will most likely continue this process of retreat until the water in the fjord becomes more shallow (Post, 1975). Click here to read more about the advance and retreat of Columbia Glacier.
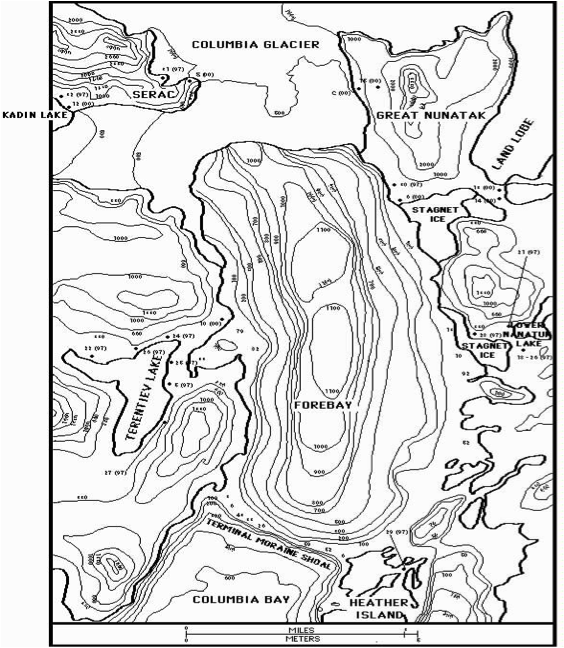
The retreat of Columbia Glacier has not only lead to our better understanding of the way tidewater glaciers experience calving, but it has also allowed glaciologists to determine when it was advancing. As the ice calves off at the margin, it exposes areas on either side of the fjord that are covered with sub-fossil trees that were run over by the glacier during its initial advance. Using dendrochronology and cross-dating, the results of recent calving by Columbia Glacier have been used as indicators of fluctuations in the past climate of Prince William Sound.
Works Cited
- Brown, C.S., M.F. Meier, and A. Post. 1982. Calving speed of Alaska tidewater glaciers, with application to Columbia Glacier. U.S. Geological Survey Professional Paper 1044-9612, p. C1-C13.
- Lapham, K.A. 2001. The past thousand years of glacial change from Columbia Glacier, Prince William Sound, Alaska. Unpublished Undergraduate Thesis – The College of Wooster.
- Mann, D.H. 1986. Reliability of a fjord glacier’s fluctuations for paleoclimatic reconstructions. Quaternary Research 25:10-24.
- Mercer, J.H. 1961. The response of fjord glaciers to changes in the firn limit. Journal of Glaciology 3(29): 850-858.
- Post, A., B. Hallet, and L.A. Rasmussen. 1998. Preliminary bathymetry of the forebay, Columbia Glacier, Alaska. Open File Report No. 4-B, sheet 2.
- Post, A. 1975. Preliminary hydrography and historic terminal changes of Columbia Glacier, Alaska. U.S. Geological Survey Hydrographic Investigations Atlas 559, 3 sheets.
- Powell, R.D. 1991. Grounding-line systems as second-order controls on fluctuations of tidewater termini of temperate glaciers, p. 75-93. In: Anderson, J.B. & Ashley, G.M. (eds.), Glacial marine sedimentation; Paleoclimatic significance. The Geological Society of America, Special Paper 261.
- Van der Veen, C.J. (ed.). 1997. Calving Glaciers: Report of a Workshop, February 28 – March 2, 1997. BPRC Report No. 15, Byrd Polar Research Center, The Ohio State University, Columbus, Ohio, 194 pages.
- Van der Veen, C.J. 1996. Tidewater calving. Journal of Glaciology 42(141): 375-385.
- Van der Veen, C.J. 1995. Controls on calving rate and basal sliding: observations from Columbia Glacier, Alaska, prior to and during its rapid retreat, 1976-1993. BPRC Report No. 11, Byrd Polar Research Center, The Ohio State University, Columbus, Ohio, 72 pages.
- Venteris, E.R. 1999. Rapid tidewater glacier retreat: a comparison between Columbia Glacier, Alaska and Patagonian calving glaciers. Global and Planetary Change 22:131-138.
- Warren, C.R. 1992. Iceberg calving and the glacioclimatic record. Processes in Physical Geography 16(3): 253-282.
Related pages
- A Thousand Year History of Glacial Change from Columbia Glacier, Prince William Sound, Alaska by Kirk Lapham
- Columbia Glacier 2001 by Clinton Bailey
- Dendroclimatology of the Columbia Bay Region, Prince William Sound, Alaska by Aaron Shear
- Late Holocene Glacial Stratigraphy of Columbia Bay Alaska: Extending the Record by Matthew Beckwith-Laube